“I wanted to work on something that didn’t exist”
In 2017 Polina Anikeeva, PhD ’09, was invited to a conference in the Netherlands to give a talk about magnetic technologies that she and her team had developed at MIT and how they might be used for deep brain stimulation to treat Parkinson’s disease. After sitting through a long day of lectures, she was struck by one talk in particular, in which a researcher brought up the idea that Parkinson’s might be linked to pathogens in the digestive system. And suddenly Anikeeva, who had pioneered the development of flexible, multifunction brain probes, found herself thinking about how she might use these probes to study the gut.
While the idea of switching gears might give some researchers pause, Anikeeva thrives on venturing beyond her academic comfort zones. In fact, the path that led to her becoming the Matoula S. Salapatas Professor in Materials Science and Engineering—as well as a professor of brain and cognitive sciences, associate director of MIT’s Research Laboratory of Electronics, and an associate investigator at MIT’s McGovern Institute for Brain Research—was rarely a clear or obvious one. There is, however, one constant in everything she does: an indefatigable curiosity that pushes her toward the edge of risk—or, as she likes to call it, “the intellectual abyss.”
After the conference in the Netherlands, she soon dove into studying the human gut, a system that doesn’t simply move nutrients through the body but also has the capacity to interpret or send information. In fact, she has come to think of it as a largely uncharted “distributed nervous system.” In 2022, she became the director of the newly launched K. Lisa Yang Brain-Body Center at MIT, where she’s directing research into the neural pathways beyond the brain—work that could shed light on the processes implicated in aging and pain, the mechanisms behind acupuncture, and the ways digestive issues might be linked not just to Parkinson’s but to autism and other conditions.
Although she hadn’t heard of it before that conference in the Netherlands, the hypothesis that piqued Anikeeva’s interest in studying the brain-body connection was first posed by the German anatomist Heiko Braak in 2003. He and colleagues posited that a type of Parkinson’s disease has environmental origins: a pathogen that enters the body through the mouth or the nasal cavity and ends up in the digestive tract,where it triggers the formation of abnormal, possibly toxic clumps of protein within neurons. That condition, known as Lewy pathology, is the hallmark of the disease.
“The reason the environmental hypothesis came about is because those Lewy bodies actually have been found in the GI tract of patients with Parkinson’s,” Anikeeva explains. “But what’s more striking is that if you go back in the medical history, Parkinson’s patients—many of them, like 80% or so—have been diagnosed with GI dysfunction, most commonly constipation, years before they get a Parkinson’s diagnosis.”
Functions, behaviors, and diseases long thought to originate in the brain might be influenced by signals from other parts of the body.
Researchers have debated the hypothesis and have yet to make definite causal connections between the ingestion of pathogens and the progression of Parkinson’s disease. But Anikeeva was intrigued.
“It’s quite controversial and it has seen some attempts at testing, but nothing conclusive,” she says. “I thought that my lab had a unique tool kit to start testing this hypothesis.”

microscopic gut-brain interfaces her team developed.
At the time, Anikeeva’s lab was focused on flexible polymer-fiber probes that can interface with the brain and spinal cord. Having developed these fibers, she and her team were testing them in mice, both to stimulate neurons and to record their signals so they could study the ways in which those signals underlie behavior. The lab had also been working on using magnetic nanomaterials to stimulate neurons so their activity could be regulated remotely—without needing to run fibers to a mouse’s brain at all.
Braak’s hypothesis made Anikeeva wonder: Could similar multifunctional probes be used to explore the digestive system? Could she and her team engineer gut-specific tools to study the neurons that make up what’s known as the enteric nervous system, which regulates sensing, moving, absorbing, and secreting—the tasks that the gastrointestinal tract must perform to digest food? And for that matter, could they study any of the body’s peripheral systems?
“I started thinking about interfacing not only with the central nervous system, but also with other organ systems in the body, and about how those organ systems contribute to brain function,” she explains.
Ultimately, this interface could help researchers understand the way the body communicates with the brain and vice versa, and to pinpoint where diseases, including Parkinson’s, originate.
“For many years neuroscience has essentially considered the brain in a vacuum. It was this beautiful thing floating, disconnected,” Anikeeva says. “Now we know that it’s not in a vacuum … The body talks back to the brain. It’s not a strictly downward information flow. Whatever we think—our personality, our emotions—may not only come from what we perceive as the conscious brain.” In other words, functions, behaviors, and neurodegenerative diseases long thought to originate in the brain—perhaps even the act of thinking itself—might be influenced by signals from other parts of the body. “Those are the signals that I’m very excited about studying,” she says. “And now we have the tools to do that.”
“It’s opened technological floodgates into these neuroscience questions,” she adds. “This is a new frontier.”
Anikeeva grew up in Saint Petersburg, Russia, the child of engineers, and showed brilliance from an early age. She was admitted to a selective science magnet school, but she briefly considered pursuing a career in art.
“I was about 15 years old when I was choosing between professional art and professional physics, and I didn’t want to be poor,” she says with a laugh. “Being good at watercolor doesn’t help with leaving Russia, which was my objective. I grew up in a very unstable political environment, a very unstable economic environment. Nobody becomes an artist if they can do something else that’s more practical.” She chose science and earned her undergraduate degree in biophysics at Saint Petersburg State Polytechnic.
But Anikeeva says her artistic brain, along with the mind-clearing avocations of climbing and long-distance running, helps her with her work today: “I use that way of thinking, the imagination, to think conceptually about how a device might come together. The idea comes first as an image.”
After graduating, Anikeeva got an internship in physical chemistry with the Los Alamos National Laboratory in New Mexico and worked on solar cells using quantum dots. In 2004, she arrived at MIT to begin her PhD in materials science and engineering.



Sirma Orguc, SM ’16, PhD ’21, in the lab.
As a graduate student, Anikeeva helped develop quantum-dot LED display technology that’s now used by television manufacturers and sold in stores around the world. She has coauthored two papers on that research with her primary advisor, Vladimir Bulović, the Fariborz Maseeh (1990) Professor of Emerging Technology, associate dean for innovation at the School of Engineering, and director of MIT.nano, and seven with Bulović and Nobel Prize winner Moungi Bawendi, MIT’s Lester Wolfe Professor of Chemistry.
But after earning her PhD in 2009, Anikeeva says, she got bored—as she frequently does. “I wanted to work on something that didn’t exist,” she says.
That led her to seek out a postdoctoral fellowship in neuroscience at Stanford University in the lab of Karl Deisseroth, one of the inventors of optogenetics, which uses laser light to activate proteins in genetically modified brain cells. Optogenetic tools make it possible to trigger or inhibit neurons in test rodents, creating an on/off switch that lets researchers study how the neurons work.
“I was really fortunate to be hired into that lab, despite the fact that my PhD, ultimately, was not in neuroscience but in optical electronics,” she says. “I saw all these animals running around with optical cables coming out of their heads, and it was amazing. I wanted to learn how to do that. That’s how I came to neuroscience.”
Realizing that the tools neuroscientists used to study complex biological phenomena in the brain were inadequate, she started to develop new ones. In Deisseroth’s lab, she found a way to improve upon the fiber-optic probes they were using. Her version incorporated multiple electrodes, allowing them to better capture neuronal signals.
Probing the brain is challenging because it’s very soft—“like pudding,” as she puts it—and the tools researchers used then were rigid and potentially damaging. So when Anikeeva returned to MIT as an assistant professor, her lab collaborated with Yoel Fink, PhD ’00, a professor of materials science and engineering as well as electrical engineering and computer science and director of MIT’s Research Laboratory of Electronics, to create very thin, highly flexible fibers that can enter the brain and the spinal cord without doing any harm (see “A Better Way to Probe the Brain,” MIT News, May/June 2015). Unlike the bulky hardware that Deisseroth was using to deliver light for optogenetics, Anikeeva’sfibers are multifunctional. They’re made of an optical core surrounded by polycarbonate and lined with electrodes and microfluidic channels, all of which are heated and then stretched in production. “You pull, pull, pull and you get kilometers of fiber that are pretty tiny,” Anikeeva explains. “Ultimately it gets drawn down to about a hair-thin structure.”
Using these ultrathin fibers, researchers can record neuronal signals and send their own signals to neurons in the brain and spinal cord of genetically engineered mice to turn them on and off. The fibers offered a new way to investigate neural responses—and earned Anikeeva a spot on our 2015 list of 35 Innovators Under 35. They also proved to be a useful therapeutic tool for drug delivery using the fibers’ microfluidic channels.
As this work hummed along, Anikeeva heard about Braak’s hypothesis in 2017 and set out to find resources to investigate the gut-brain connection. “I promptly wrote an NIH grant, and I promptly got rejected,” she says.
But the idea persisted.
Later that year, neural engineers studying brain interfaces at Duke invited Anikeeva to give a talk. As she had gotten in the habit of doing during her travels to other universities, she looked up researchers working on GI systems there. She found the gut-brain neuroscientist Diego Bohórquez.
While the brain is extraordinarily complex, from an engineering and research standpoint it’s much more convenient to study than the digestive tract.
“I told him that I’m really interested in the gut, and he told me that they were … studying nutrient absorption in the gut and how it affects brain function,” Anikeeva recalls. “They wanted to use optogenetics for that.”
But the glass fibers he’d been trying to use for optogenetics in the gut could do serious damage to the fragile GI system. So Anikeeva proposed a trade of sorts.
“I thought that we can easily solve Diego’s problems,” she says. “We can make devices that are highly flexible, basically in exchange for Diego teaching us everything about the gut and how to work in that really fascinating system.”
Bohórquez remembers their first meeting, the beginning of a fruitful collaboration, in some detail. “She said, ‘I see that you are doing some really interesting work in sensations and the gut. I’m sure that you’re probably trying to do something with behavior,’” he says. “And then she pulls out these fibers and said, ‘I have this flexible fiber. Do you think that you can do something with it?’”
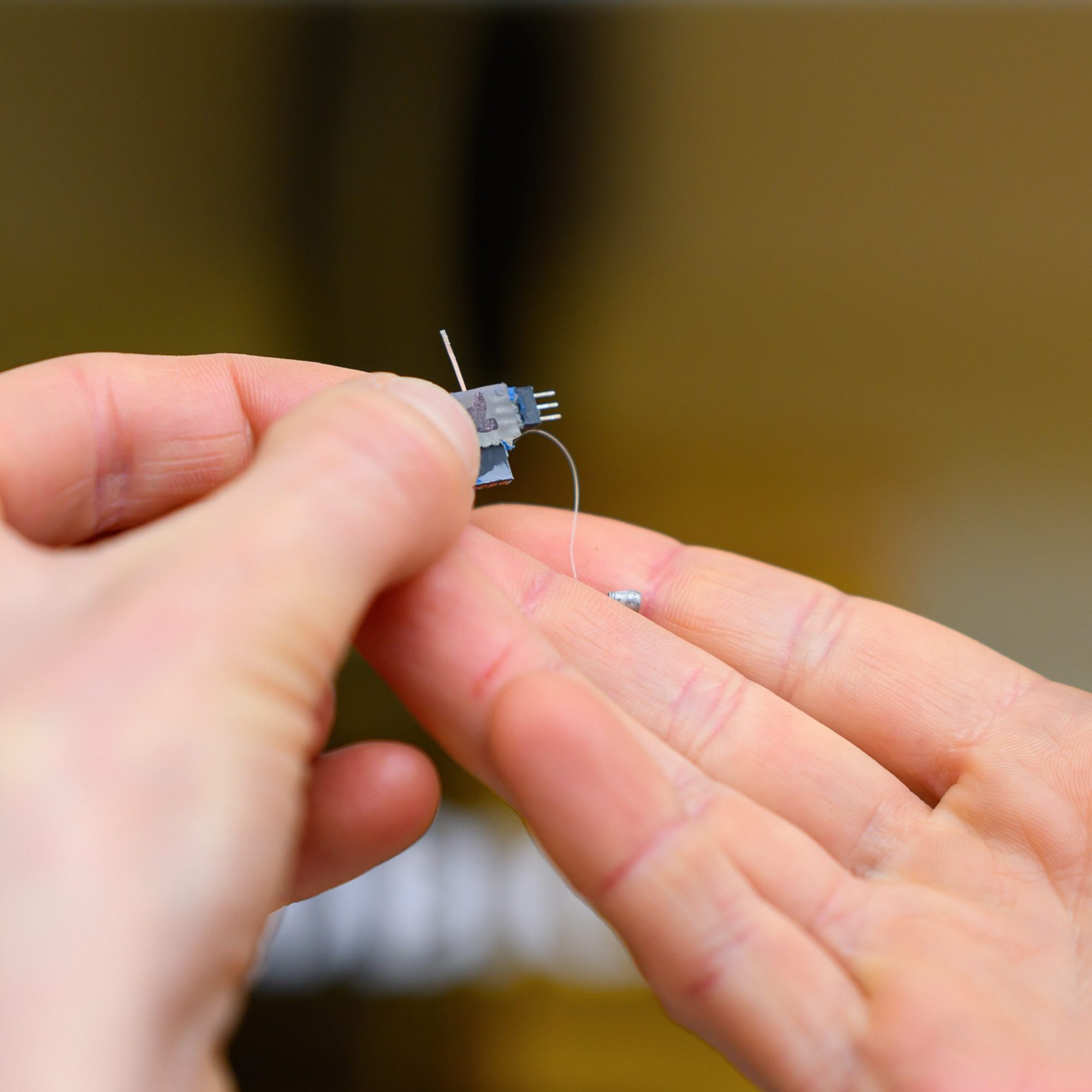
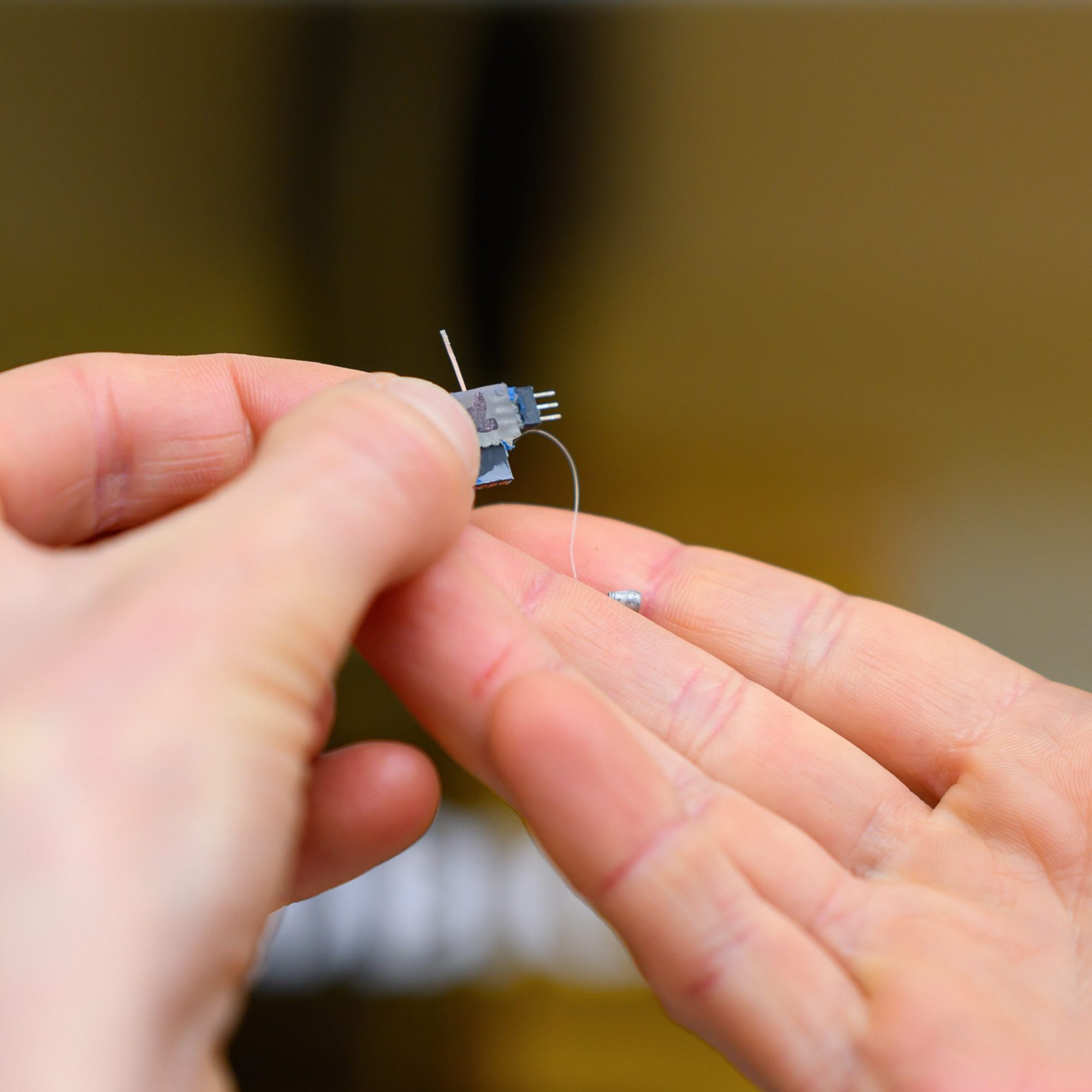
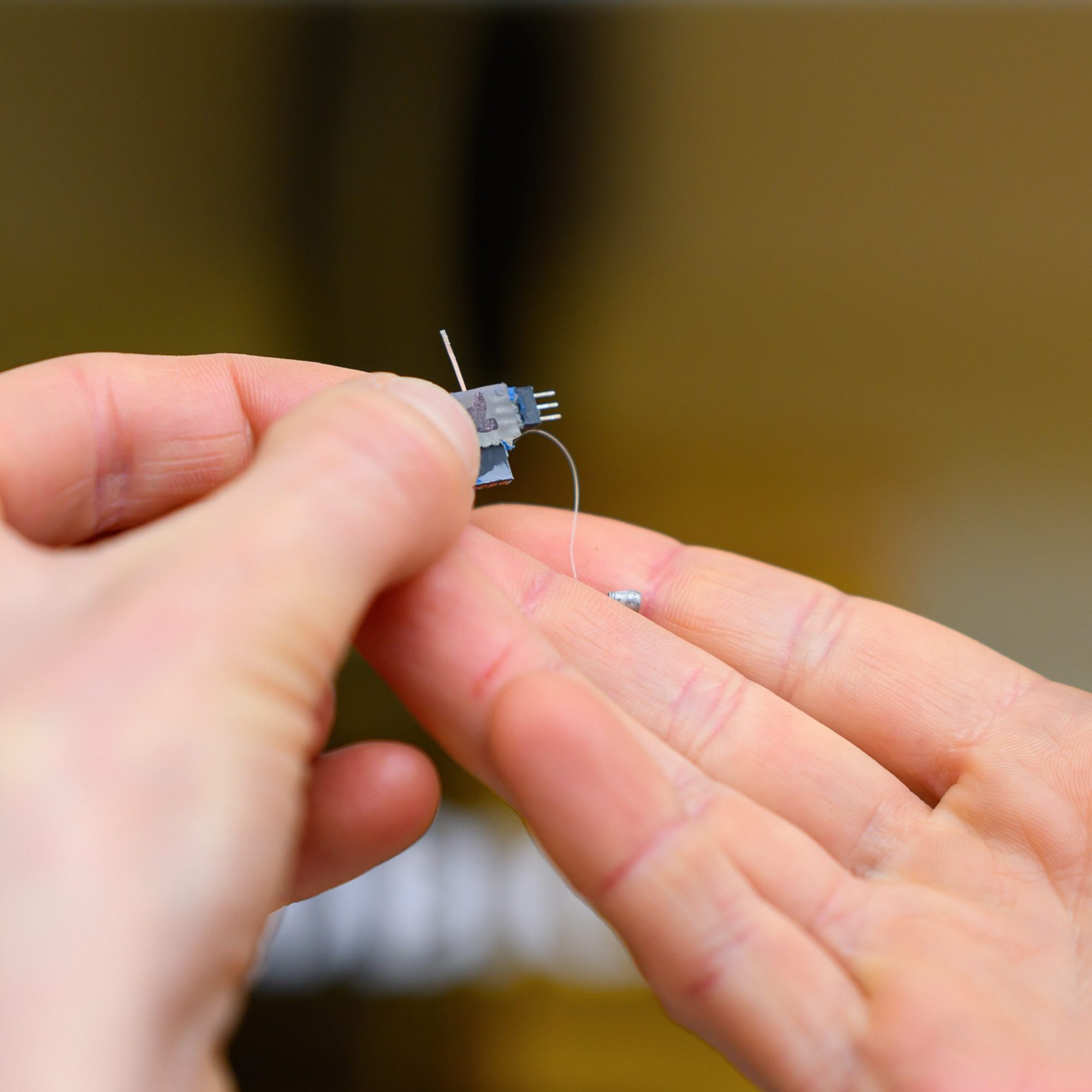
interface.



stretchable organ probes under a microscope.
She returned to MIT and, she says, began to “take this lab that is a rapidly moving aircraft carrier and start reorienting it from working on the brain to working on the gut.”
The move may have surprised colleagues, but Anikeeva refuses to do anything if it loses her interest—and while the brain is extraordinarily complex, from an engineering and research standpoint it’s much more convenient to study than the digestive tract. “The gut wall is about 300 microns or so,” Anikeeva says. “It’s like three to four hairs stuck together. And it’s in continuous motion and it’s full of stuff: bile, poop, all the things.” The challenges of studying it, in other words, are nothing short of daunting.
The nervous system in the gut, Anikeeva explains, can be thought of as two socks, one inside the other. The one on the outside is the myenteric plexus, which regulates peristalsis—the rhythmic contraction of muscles that enables food to move along the gastrointestinal tract, a process known as motility. The one on the inside is the submucosal plexus, which is closer to the mucosa (the mucus-coated inner lining) and facilitates sensing within the gut. But the roles of the plexuses are not fully understood. “That’s because we can’t just implant the gut full of hardware the same way we do in the brain,” Anikeeva says. “All the methods, like optogenetics and any kind of electrical physiology—all of that was pretty much impossible in the gut. These were almost intractable problems.”
Anikeeva’s work developing tools for the brain had been so successful and groundbreaking that it was difficult for her to find financial support for her pivot to other parts of the body. But then, she says, came “another fateful meeting.”
In 2018, she gave a presentation at a McGovern Institute board meeting, conveying her latest ideas about studying Parkinson’s disease and engineering tools to explore the GI system. Lisa Yang, a board member, mentioned that many people with autism also suffer from GI dysfunction—from motility disorders to food sensitivities. Yang was already deeply interested in autism, and she and her husband had just launched the McGovern Institute’s Hock E. Tan (’75, SM ’75) and K. Lisa Yang Center for Autism Research the year before.
“She was interested in this gut-brain connection,” Anikeeva remembers. “I was brought into the Center for Autism Research for a small project, and that small project kind of nucleated my ability to do this research—to begin developing tools to study the gut-brain connection.”
As that effort got underway, a number of colleagues at MIT and elsewhere who were also interested in brain-body pathways were drawn to the new research.



“As our tools started to mature, I started meeting more people and it became clear to me that I’m not the only person interested in this area of inquiry at MIT,” she says. “The tools opened this frontier, and the Brain-Body Center bubbled up from that.”
To launch into their work on the gut-brain connection, Anikeeva and her team had to completely rethink the fibers they had designed previously to study the brain.
In brain probes, all the functional features sit at the tip of the fiber, and when that fiber is threaded into the skull, the light-emitting tip faces downward, allowing researchers a view of everything under it. That doesn’t work with the GI system. “It’s not how you want to interface with the gut,” Anikeeva says. “The gut is a lumenal organ—it’s a sock—and the nervous system is distributed in the wall.”
In other words, if the probe is looking downward, all it will see is matter passing through the gut. To research the GI tract, Anikeeva and her colleagues needed these features to sit laterally, along the length of the fiber. So with this fabrication challenge in mind, Anikeeva again approached Fink, a longtime mentor and collaborator—and a fellow TR35 veteran.
Mice “would normally eat ferociously” when given access to food after fasting. “But if you stimulate those cells in the gut, they would feel full.”
Together they developed a way to distribute microelectronic components—LEDs for optogenetic stimulation, temperature sensors, and microfluidic channels that can deliver drugs, nutrients, and genetic material—along the fiber by essentially creating a series of pockets to contain them. Grad student Atharva Sahasrabudhe put in countless hours to make it happen and optimized the process with the help of technician Lee Maresco, Anikeeva says. Then, with Anantha P. Chandrakasan, dean of MIT’s School of Engineering, the Vannevar Bush Professor of EECS, and head of MIT’s Energy-Efficient Circuits and Systems Group, the team designed a wireless, battery-powered unit that could communicate with all those components.
The result was a fiber, about half a millimeter by one-third of a millimeter wide, made out of a rubbery material that can bend and conform to a mouse’s gut yet withstand its harsh environment. And all the electronic components housed within it can be controlled wirelessly via Bluetooth.
“We had all the materials engineers, and then we collaborated with our wireless colleagues, and we made this device that could be implanted in the gut. And then, of course, similar principles can also be used in the brain,” Anikeeva explains. “We could do experiments both in the brain and the gut.”



In one of the first experiments with the new fibers, Anikeeva worked with Bohórquez and his team, who had determined that sensory cells in the GI tract, called neuropods, send signals to the brain that control sensations of satiety. Using mice whose cells are genetically engineered to respond to light, the MIT and Duke researchers used the specialized fibers to optically stimulate these cells in the gut.
“We could take mice that are hungry, that have been fasting for about 18 hours, and we could put them in a cage with access to food, which they would normally eat ferociously,” Anikeeva says. “But if you stimulate those cells in the gut, they would feel full even though they were hungry, and they would not eat, or not as much.”
This was a breakthrough. “We knew that the technology works,” she says, “and that we can control gut functions from the gut.”
Next Anikeeva’s team wanted to explore how these neural connections between the gut and the brain can influence a mouse’s perception of reward or pleasure. They put the new fiber into the area of the brain where reward perception is processed. It’s packed with neurons that release dopamine—the “happy hormone”—when activated.
Then they ran tests in which mice had a choice between two compartments in a cage; each time a mouse entered a particular one, the researchers stimulated its dopamine neurons, causing the mouse to prefer it.
To see if they could replicate that reward-seeking behavior through the gut, the researchers used the gut-specific fibers’ microfluidic channels to infuse sucrose into the guts of the mice whenever they entered a particular compartment—and watched as dopamine neurons in the brain began firing rapidly in response. Those mice soon tended to prefer the sucrose-associated compartment.
But Anikeeva’s group wondered if they could control the gut without any sucrose at all. In collaboration with Bohórquez and his team at Duke, the researchers omitted the sucrose infusion and simply stimulated the gut neurons when the mice entered a designated compartment. Once again, the mice learned to seek out that compartment.
“We didn’t touch the brain and we stimulated nerve endings in the gut, and the mice developed the exact same type of preference—they felt happy just when we stimulated the nerve endings in their small intestines using our technology,” Anikeeva says. “This, of course, was a technical demonstration that it is now possible to control the nervous system of the gut.”
The new tools will make it possible to study how different cells in the gut send information to the brain, and ultimately the researchers hope to understand the origins not only of digestive diseases, like obesity, but of autism and neurodegenerative diseases such as Parkinson’s.
Researchers at the Brain-Body Center are already exploring those connections. “We’re particularly interested in the gut-brain connection in autism,” Anikeeva says. “And we’re also interested in more affective disorders, because there is a big genetic link, for instance, between anxiety and IBS [or irritable bowel syndrome].”
In the future, the technology also could lead to new therapies that can control gut function more precisely and effectively than drugs, including semaglutides like Ozempic, which have made headlines in the past year for weight control.
Now that Anikeeva has developed and tested the device in the GI system and solved a lot of technical challenges, other peripheral systems in the body could be next.
“The gut is innervated, but so is every organ in the body. Now we can start asking questions: What is the connection to the immune system? The connection to the respiratory system?” she says. “All of these problems are now becoming tractable. This is the beginning.”
Probing the mind-body connection
Founded in 2022, the K. Lisa Yang Brain-Body Center at MIT is focusing on four major lines of research for its initial projects.



GUT-BRAIN:
Polina Anikeeva’s group is expanding a toolbox of new technologies and applying these tools to examine major neurobiological questions about gut-brain pathways and connections in the context of autism spectrum disorders, Parkinson’s disease, and affective disorders.



AGING:
CRISPR pioneer Feng Zhang, the James and Patricia Poitras Professor of Neuroscience at MIT and an investigator at the McGovern Institute, is leading a group in developing molecular tools for precision epigenomic editing and erasing accumulated “errors” of time, injury, or disease in various types of cells and tissues.



PAIN:
The lab of Fan Wang, an investigator at the McGovern Institute and professor of brain and cognitive sciences, is designing new tools and imaging methods to study autonomic responses, activity of the sympathetic and parasympathetic neurons, and interactions between the brain and the autonomic nervous system, including how pain influences these interactions.



ACUPUNCTURE:
Wang is also collaborating with Kelly Metcalf Pate’s group in MIT’s Division of Comparative Medicine, to advance techniques for documenting changes in brain and peripheral tissues induced by acupuncture in mouse models. If successful, these techniques could help make it possible to better understand the mechanisms involved in acupuncture—specifically, how the treatment stimulates the nervous system and restores function.
Part of the goal of the Brain-Body Center, Wang says, is to dissect how the circuits of the central nervous system interact with the peripheral autonomic system to generate emotional responses to pain. She says her research has led her to a deeper understanding of the two responses to pain—sensory and emotional. The latter, a function requiring the autonomic nervous system, is what leads to a sense of suffering. If researchers can prevent the autonomic responses elicited by pain, she explains, then the same stimulus may produce “a sensation without pain.” The idea is to develop devices to manipulate autonomic responses in mice, and then ultimately develop devices that can help humans. —Julie Pryor and Georgina Gustin